This concept relies on controlling magnetism using a gate voltage, which is not only used to generate charge accumulation at the surface of the magnetic materials, but also to move ions inside a neighbouring oxide layer. This produces large changes in the magnetic properties which are also non-volatile, since the effects are related to ionic rearrangement and bond formation (oxidation/reduction) under voltage. Non-volatility and a large amplitude of the gating effects is what makes magneto-ionics particularly attractive for spintronics applications, where dynamic reconfiguration of magnetic properties can be of great interest.
This emerging field is currently one of the most promising paths towards low-power consumption in spintronics memory applications. Gating could be used to reduce the switching currents needed to write information without compromising the memory’s thermal stability. In ferromagnetic nanostructures based on CoFeB and Co ultra-thin films, materials used in new storage technologies like STT-RAM, it has been demonstrated that an interface with an ionic conductor can be tailored chemically by ionic motion, if a gate voltage is applied across the structure, creating new chemical bonds at the interface, changing, for example, the degree of oxidation. This process can boost perpendicular magnetic anisotropy (PMA) and promote ion intercalation deep inside the magnetic materials, modifying its coupling with the substrate. Magneto-ionics gating can also greatly modify parameters like the Dzyaloshinskii Moriya interaction (DMI), as first demonstrated by our group, which is crucial for the control of magnetic chiral structures as skyrmions.
Fig. 1: Magneto-ionic devices have two binary states, each can be defined as ‘weak’ or ‘strong’ easier or harder to switch) thanks to non-volatile ionic motion (circles) under a gate voltage.
Magneto-ionics in Pt/Co/HfO2
This work was an extended collaboration between laboratories in France and abroad (Germany, Italy, USA, Japan). We could show that ionic liquid gating could permanently change not only the magnetic anisotropy but also the Dzyaloshinskii-Moriya interaction (DMI) in Pt/Co, a material with a large potential for practical applications in spintronics. The DMI is a key ingredient for the observation of chiral magnetic structures such as skyrmions, and its dynamic and nonvolatile control is a key aspect of the development of magneto-ionic functionalities for applications in spintronics. We could show the gating effect on DMI by means of Brillouin Light Scattering and its effect on domain wall dynamics. We also provided an extensive chemical and structural characterisation of the system before and after gating.
Fig. 2: Cartoon of the device, and hysteresis loops measured in a perpendicular magnetic eld for an as-grown lm and gated samples. (inset) Magnetic domains after gating. (b) DW motion experiments in as-grown and gated samples and variation of the D parameter as a function of the gating time.
These gate-induced changes are related to the migration of oxygen species from HfO2 into the Co andPt layers, producing a gentle oxidation of the Co (observed by XPS and XAS) and important structural changes at the interfaces (measured by PNR). Oxidation at the Co/HfO2 interface is thought to be responsible for the variations induced to the PMA, while Co oxidation and ion intercalation at the Pt/Co interface is thought to lead to a decoupling between the Pt and Co layers and, in turn, a weakening of the DMI. Our results showed a complex scenario wherein gating induces structural, chemical, and magnetic changes across the multilayer, indicating the role of ionic migration across multiple interfaces.
Multiple magneto-ionic regimes in Ta/CoFeB/HfO2
Subsequently we focused on CoFeB-based stacks, motivated by their great technological potential and our deep knowledge of their structure and magnetic properties, and investigated the Ta/CoFeB/HfO2 system by ionic liquid gating. In these stacks we found that a gate voltage drives, in a nonvolatile way,the system from an underoxidized state exhibiting in-plane anisotropy (IPA) to an optimum oxidation level resulting in PMA and further into an overoxidized state with IPA, this is shown in the hysteresis loops presented in Fig. 3 (left).
The IPA to PMA transition, named regime I, is found to be significantly faster than the PMA to IPA transition, named regime II. Moreover, regimes I and II show very different reversibility behaviors. The reversibility of the effects of a gate voltage of -2 V has been tested with positive gate voltages going up to +4 V in regimes I and II. The effects of the application of +4 V for 10min and up to 1 hour to a magnetic state close to PMA in regime I are shown in Fig. 3 (right), where only minor changes are induced in the magnetic
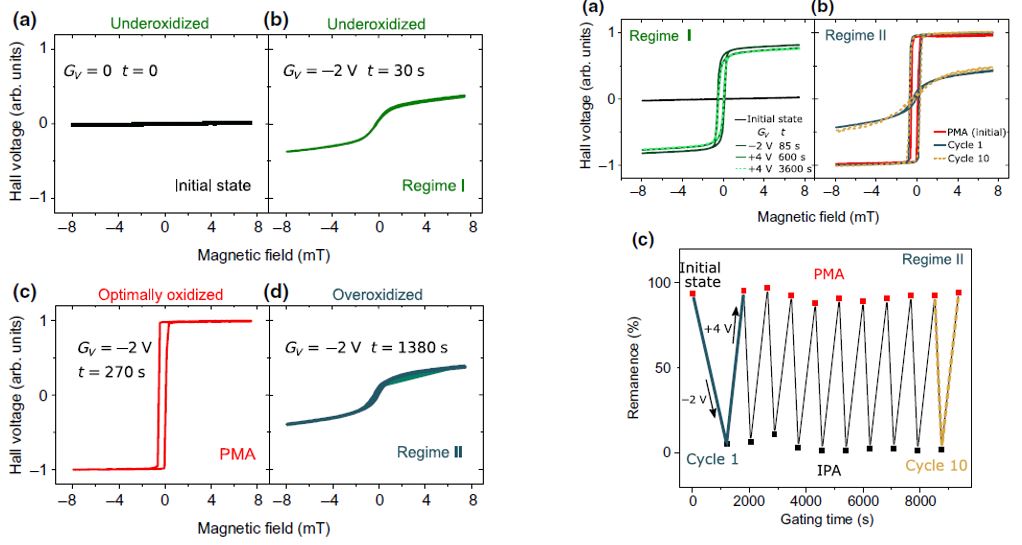
Fig. 3: (left) Hysteresis loops under gate voltage for dierent gating times. (right) Suppressed reversibilityof the IPA to PMA transition under GV = +4 V in regime I, and full reversibility in regimeII. The remanence as a function of gating time plot shows reversibility over 10 cycles.
state. The same eect is found for other intermediate states in regime I, positive gate voltages up to +4 V and long exposures cannot induce a recovery of the initial under oxidized state. Regime II shows an entirely different behavior; a fully reversible transition between IPA and PMA can be observed. Fig. 3 (right) shows the Hall voltage hysteresis loops corresponding to reversibility cycles number 1 and 10, and the fully reversible remanence variation of the entire series.
The existence of two magneto-ionic regimes, with different degrees of distinct reversibility, has been linked to a difference in the chemical environment of the anchoring points of oxygen species added to underoxidized or overoxidized layers. That is, reversibility depends on how much oxygen already exists in the layer before gating. Therefore, our results showed that multiple magneto-ionic regimes can exist in a single device, which makes the optimisation of magneto-ionic reversibility a far more complex task than simply identifying materials (oxides, interfaces) as reversible or non-reversible. The results presented here reveal the complexity of magneto-ionics and the importance of a deep understanding of the ionic mechanisms involved in order to design robust and reliable devices for spintronics applications.
What about magneto-ionics using MgO?
Advanced magnetic memory technologies rely on magnetic tunnel junctions for the reading operation,which typically contain the following layer arrangement: CoFeB/MgO/CoFeB. Here the quality of the MgO layer is of paramount importance since the read-out operation depends on the efficient spin transport(tunneling magneto resistance) through it. We therefore investigated the system CoFeB(Pt)/MgO/HfO2, where a small amount of Pt was introduced at the interface (0.09 nm) as a seed for the crystalline growth of MgO. The HfO2 ionic reservoir was placed on top of the MgO layer. In this system the MgO layer was found to be polycrystalline, unlike the HfO2 layer, which is amorphous. This changes significantly the scenario of ionic difusion, which in the previous systems can happen within an amorphous medium and here most likely happens through the grain boundaries of the
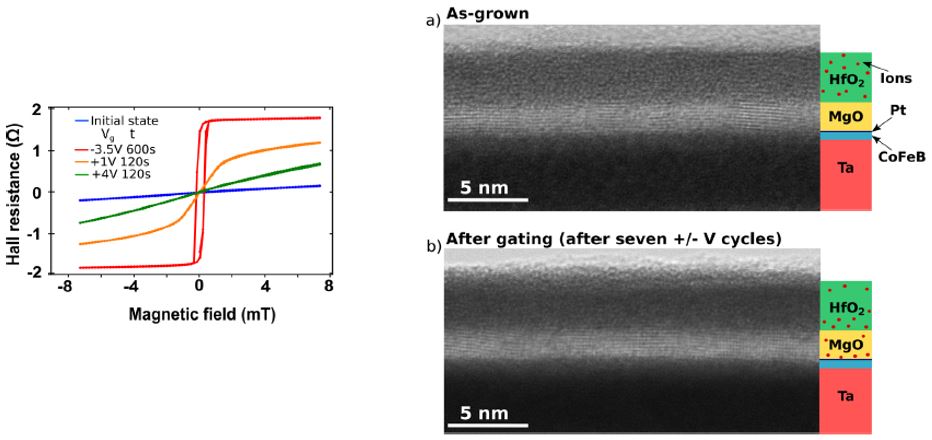
Fig. 4: (left) AHE hysteresis loops for Ta/CoFeB(Pt)/MgO/HfO2 where magneto-ionic gating inducesa spin-reorientation transition. (right) TEM images of samples as-grown and after seven cycles ofapplication of -3.5 V/+4 V gate voltages.
MgO. Nevertheless, we could show that magneto-ionic gating is also very effective in this system, despite its crystalline structure. Fig.9 (left)shows anomalous Hall effects hysteresis loops showing that a spin reorientation transition can be obtained through gating. Moreover, we conducted transmission electron microscopy measurements (TEM)in the as-grown state and after seven cycles of gating which showed that the polycrystalline structure of the MgO is not affected by the gating process.
This is a very important result, showing that effective magneto-ionic gating can be conducted through the MgO layer without degrading its structural properties, which is extremely important for devices where a good quality CoFeB/MgO interface is a must. Moreover, we were able to show that the crystalline volume of the MgO actually increases under gating, and that magneto-ionic cycling can make the system evolve irreversibly towards an equilibrium regime, were the magneto-ionic effects are fully reversible. This shows how changes in structural properties can lead to irreversible effects that can combine with reversible effects from the ionic diffusion at the oxide/ferromagnetic interface. In addition, in this system we were able to compare the magneto-ionic performance in ionic liquid devices with that of solid-state devices, confirming that both device designs produce the same effects. In solid-state devices we could clearly show the effects of both reversible and irreversible magneto-ionic effects as shown in Fig. 5.
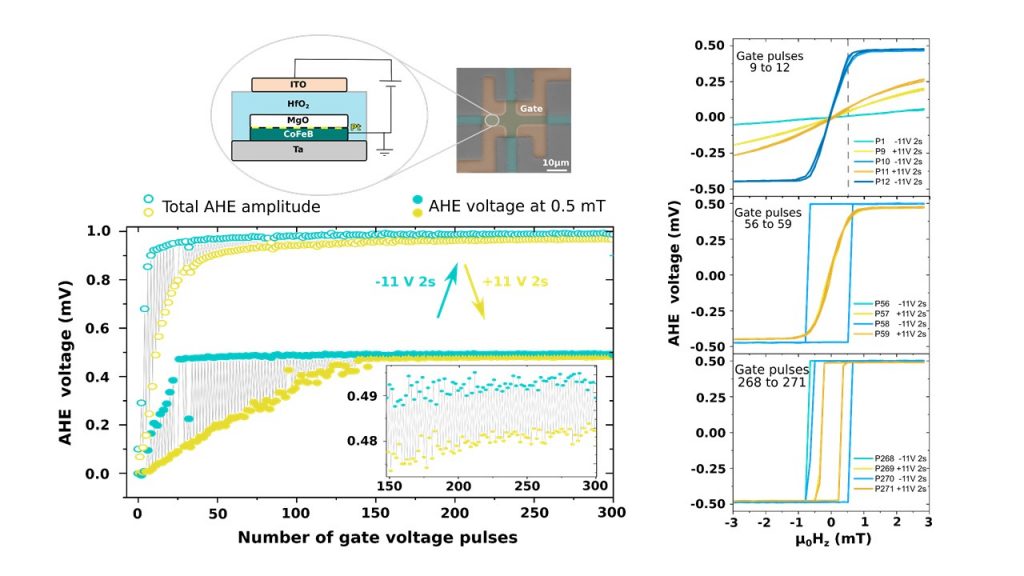
Fig. 5: Performance of solid-state devices over extended cycling. The irreversible effects coming from the chage in crystalline structure of the MgO are combined with reversible effets of ionic diffusion at the CoFeB/MgO interface.
Magneto-ionics in multi-repeat layer and 90° PMA-to-IPA domain walls
Recently, we expanded our research to magnetic multilayers in collaboration with Laboratoire Albert Fert in Palaiseau. Traditionally, magneto-ionics modifies interfacial properties in thin films with a single magnetic layer. However, multi-layer structures provide unique advantages for device functionality. By strategically layering repetitive sequences of magnetic and non-magnetic layers we can effectively tune both PMA and DMI. This concept leverages the strong interface contributions and the combined effects of successive interfaces and holds promise for designing materials where DWs and skyrmions can be electrically controlled, a crucial step toward advanced spintronics devices.
We investigated the magneto-ionic modulation of PMA in multi-repeat Pt/Co/Al layers. We demonstrated a reversible IPA to PMA non-volatile transition in the topmost Co layer using a gate voltage.For repetitions with n = 2 and n = 4, this enables control over the total number of active PMA magnetic layers, denoted as m, shifting between m = n and m = n+1 layers. We also showed that the gate-voltage induced spin-reorientation transition PMA to IPA occurs through the propagation of a single magnetic domain wall, this time not between two perpendicular states but between two magnetic anisotropy regions, a spin structure that has never been manipulated before. Fig. 6 (left) illustrates the stack
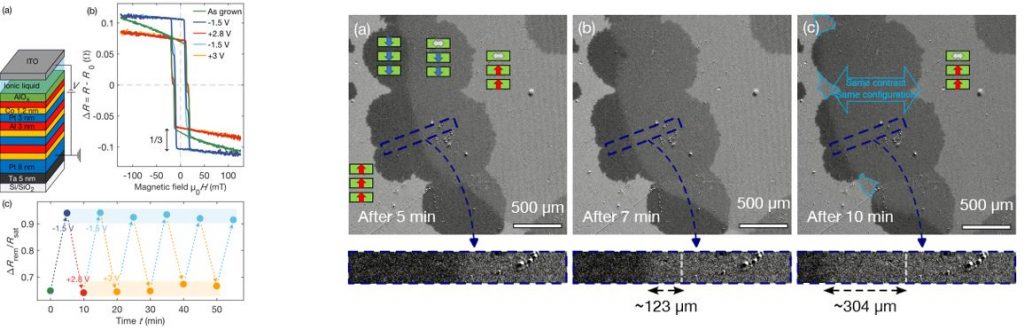
Fig. 6: (left) Graphic representation of a multilayer stack of Ta/Pt/[Co/Al/Pt]2/Co/Al/AlOx , and anomalous Hall resistance measured in the as-grown state and after gating. The lower panel shows the good reversibility of the system. (right) Kerr microscopy images of the domain conguration during the application of a gate voltage of +2.5 V over time. The bottom insets show a moving domain wall between PMA and IP magnetic anisotropy domains in the topmost Co layer.
with n=3, where the application of a gate voltage of -1.5 V during 5 min induces a spin-reorientationtransition of the magnetization of the topmost Co layer towards PMA. It transforms the quasi-linear variation of the anomalous Hall resistance R with the external out-of-plane magnetic field into a sharp switch. Moreover, the switching accounts for about two-thirds of the total amplitude of the R signal,indicating that the switching of the topmost Co magnetization in its gate-induced PMA state is coupled to the switching of magnetization in the middle Co layer. Subsequently, a gate voltage of +2.8 V is applied during 5 min, reverting the topmost Co magnetization back into an IPA state. For both positive and negative gate voltages, the anisotropy of the two buried Co layers remains seemingly unchanged. The good reversibility and cyclability of these effects are presented in Fig. 6 (left).
The dynamics of the gate-induced PMA to IPA spin reorientation transition in the topmost Co layer has been imaged in real-time using Kerr microscopy. Initially, the sample is set to the PMA state, where magnetic domains are nucleated in the three Co layers of the multilayer by applying a negative magnetic field. Then, a gate voltage of +2.5 V is applied during a total gating time of 10 min. In Fig. 6 (right), Kerr microscopy images are shown, measured through the transparent ionic liquid – ITO gate while applying the +2.5 V gate voltage to the stack for gating times: 5 min (a), 7 min (b), and 10 min (c). A pronounced magnetic contrast between merged circular structures and the background is observed, corresponding to the PMA (up and down) domains nucleated using magnetic fields. A subtler contrast change propagates from right to left, making the swept areas appear in a lighter color, regardless of the presence of PMA magnetic domains. Four contrasts are observed in 10 (right, a-c), indicating that the domains remain in the bottom Co layer independently of the IPA or PMA character of the topmost Co layer. The corresponding magnetic configurations are schematized in panels (a) and (c). This voltage-gated induced moving front moves from right to left with a velocity of approximately 61 m/min, as shown in the insets.
These measurements demonstrate that the gate-driven transition between PMA and IPA occurs through the creation of a single 90° domain wall between the PMA and IPA regions, propagating across the top Co layer. The motion of this DW can be precisely controlled using the gate-voltage, and its position remains fixed once the gate-voltage is turned off. This opens the way to designing spintronic devices that exploit all-electrical and ultra-low power control of the propagation of PMA to IPA DWs. In particular, the electrical control of the nucleation and position of these DWs could lead to new functionalities in magneto-logic devices and provides interesting perspectives for the study and optimization of the dynamics of magneto-ionic effects.